
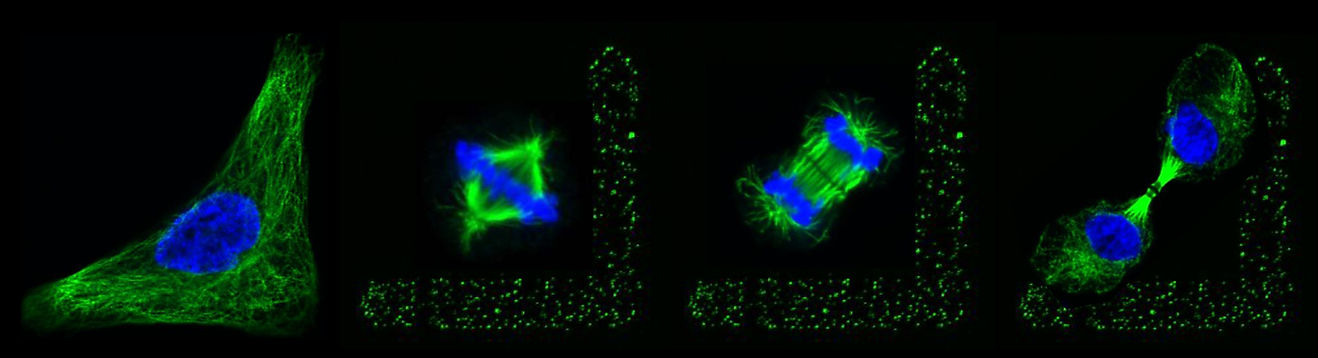
Our lab is interested in dissecting the mechanisms of a few fundamental processes. The description of these processes and our interest is mentioned below.
1) Understanding the mechanisms of spindle orientation
Cell division is an essential process for the maintenance of life, required for the development during embryogenesis and adult life forms. In metazoans, somatic cells divide by the process of mitosis. During mitosis, a diamond shape microtubule-based structure is responsible for the alignment of the mitotic spindle at a particular orientation in X, Y, and Z axes of a cell, as well as chromosomes segregation. The correct orientation of the mitotic spindle during metaphase dictates the future plane of the cell division. In human cells, the proper spindle orientation during metaphase is governed by an evolutionarily conserved membrane-anchored complex known as the ternary complex. The ternary complex is composed of NuMA/LGN/Gαi in humans and LIN-5/GPR-1/2/Gα in Caenorhabditis elegans. It is well established that NuMA, an evolutionarily conserved protein, is associated with the minus-end-directed microtubule-associated protein complex dynein at the cell membrane. It is the dynein motor activity on the astral microtubules that is responsible for the cortical force generation and thus for the alignment of the mitotic spindle [Figure 1]. Despite the in-depth knowledge acquired over the year about the functioning of the ternary complex and dynein in spindle orientation, the molecular mechanisms that modulate the spatiotemporal dynamics of this complex during mitosis remain elusive. In the context of spindle orientation, we like to understand how the cortical levels of the ternary complex and dynein are spatiotemporally-regulated during mitosis.
Figure 1:Cortically anchored ternary complex control spindle positioning in animal cells. Working model of spindle positioning in human cells. The ternary complex (NuMA/LGN/Gαi) is anchored below the cell cortex and recruits dynein motor protein complex. Dynein being a minus-end-directed microtubule-dependent motor complex attempts to move towards the centrosomes (shown by light brown arrow), but since it is anchored, it instead pulls the astral microtubules resulting in the generation of the pulling forces towards the cell cortex (shown in black arrow).
2) Unraveling the mechanisms of polarity establishment in animal cells
Proper cell polarity establishment is critical for development and stem cell lineages. Polarity establishment is dependent on accurate contractility of the actin cytoskeleton at the cell cortex below the plasma membrane. Any error in the polarity establishment can lead to defects during the development and self-renewal of the stem cells. In the one-cell stage of C. elegans embryo centrosome, the localized signal is critical for regulating polarity establishment in the one-cell embryo. It has been suggested that pericentriolar material (PCM) proteins of the centrosome are needed for polarity establishment. Furthermore, microtubules are also linked in setting up the polarity in the one-cell embryo. It is hypothesized that centrosome initiate polarity establishment by locally inhibiting actomyosin contractions in its vicinity (Figure 2). Mutants that are defective for actomyosin-based contractility are impaired in establishing polarity. This event leads to the formation of the defective partition of the evolutionarily conserved [PAR] protein. We are interested in understanding the mechanisms by which centrosomes-based component/s locally perturb actomyosin network within its vicinity and initiates anisotropy for proper polarity establishment.
Figure 2: Cortical plane images from the time-lapse confocal microscopy of embryo coexpressing GFP-NMY-2 (a marker for actomyosin network) and mCherry-PAR-2 (polarity marker) in control embryo. Time is represented in minutes, with t=0 corresponding to the frame that is approximately the time of polarity establishment. In control embryo the GFP-NMY-2 foci move from the posterior (P) to the anterior surface (A) that coincide with the appearance of the mCherry-PAR-2 at the cortical surface. The Kymograph analysis from time-lapse confocal microscopy of embryos expressing GFP-NMY-2 and mCherry-PAR-2 in control is shown on the right.

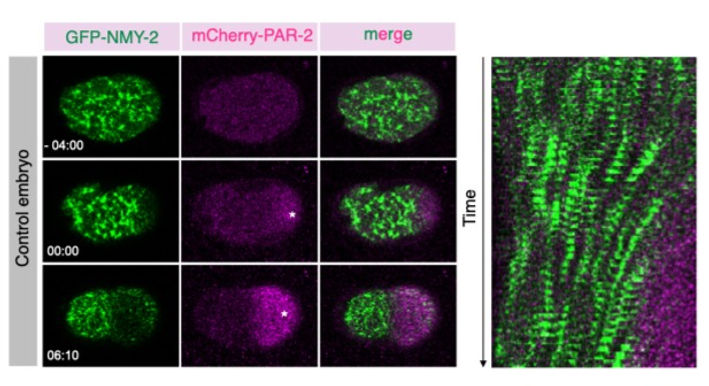